Program
The online participant can join the meeting with VooV Meeting/腾讯会议,
Conference theme: ICVFM2023 NanJing Oct16-19
Meeting time: October 17, 2023, 8:00-18:00 (GMT+08:00) China Standard Time – Beijing
Recurrence period: from 8:00 to 18:00 on October 17, 2023 to October 19, 2023, daily
Click on the link to join or add to the meeting list:
https://meeting.tencent.com/dm/t5pLgtOf5z3z
Conference No.: 715-1281-5773
Conference password: 1952
We look forward to seeing you in the conference!
Schedule of Events
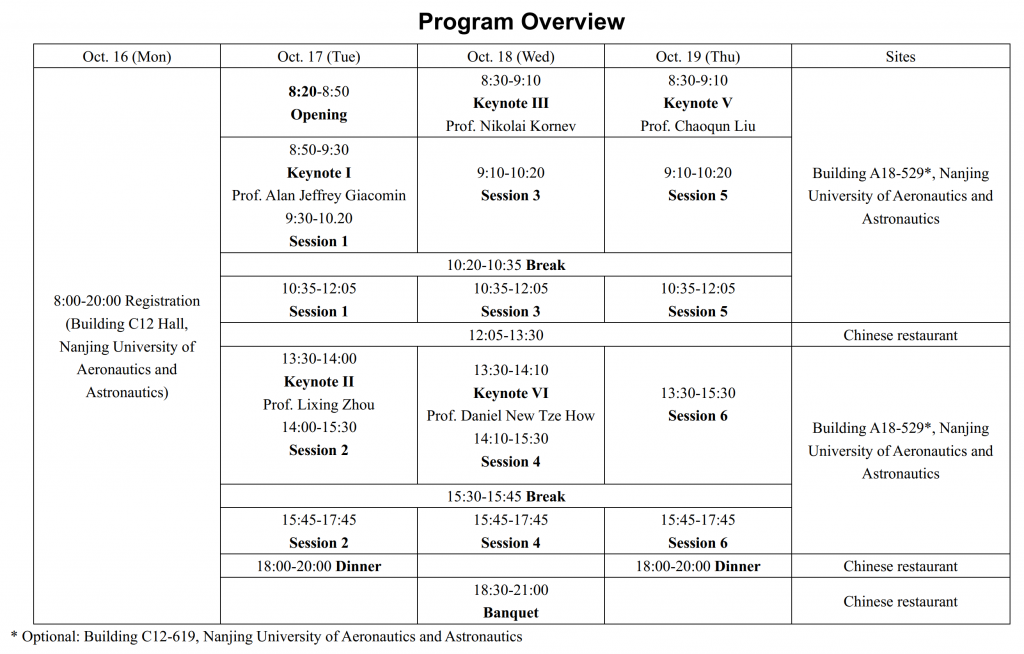
Program(updated on Oct 12th)
Monday, Oct. 16, 2023 | ||
Time | Activity | Address |
8:00-20:00 | Registration | Building C12 Hall, Nanjing University of Aeronautics and Astronautics |
18:00-20:00 | Reception | Chinese restaurant |
Tuesday, Oct. 17, 2023 | ||||
Building A18-529, Nanjing University of Aeronautics and Astronautics | ||||
Time | Activity | Chair: Prof. Xiande Fang, Nanjing University of Aeronautics and Astronautics, China | ||
8:20-8:50 | Opening | Vice-President,Prof. Bin Jiang, Nanjing University of Aeronautics and Astronautics, China | ||
Morning, Chair: Prof. Nikolai Kornev, University of Rostock, Germany | ||||
Time | Activity | PaperNo. | Corresponding author | Title |
8:50-9:30 | Keynote speech | K01 | Alan Jeffrey Giacomin (Queen’s University, Canada) | Recent Advances in Polymer Viscoelasticity from General Rigid Bead-Rod Theory |
9:30-9:45 | Oral | 008 | Leslie K. Norvihoho, Jing Yin, Shuyan Chen, Dongqing Zhu, Zhifu Zhou, Bin Chen (Xi’an Jiaotong University, China) | Numerical study on the dispersion of human expectorated cough droplets with seasonal influenza in an office |
9:50-10:05 | Oral | 042 | Xiaohuan Li, Xiande Fang (Nanjing University of Aeronautics and Astronautics, China) | Analysis of wall temperature hysteresis in pool boiling experiment under normal-gravity and hypergravity |
10:05-10:20 | Oral | 071 | Long Xia, Weijian Chen, Qianlong Ding, Hao Shen, Dong Shen (Nanjing University of Aeronautics and Astronautics, China) | Analysis of the influence of dynamic characteristics of large droplets on collection coefficient |
10:20-10:35 | Break | |||
Chair: Prof. Huixiong Li, Xi’an Jiaotong University, China | ||||
10:35-10:50 | Oral | 006 | Tong Zhao, Yalei Bai, Chuanyu Gao, Lei Ding ,Wangbo Wang (Nanjing University of Aeronautics and Astronautics, China) | Numerical investigation of flow separation control over an hump airfoil using sweeping jet actuator |
10:50-11:05 | Oral | 047 | Zhiqiang He , Xiande Fang , Xinyi Wang , Yeqi Qin (Nanjing University of Aeronautics and Astronautics, China) | Evaluation analysis of correlations of heat transfer coefficient of saturated flow boiling in helically coiled tubes |
11:05-11:20 | Oral | 069 | Chaowu Xiang, Weijian Chen, Zekun Mei, Shuai Xu, Penglei Zhang (Nanjing University of Aeronautics and Astronautics, China) | Modeling and verification of plate fin heat exchanger |
11:20-11:35 | Oral | 041 | Jieyu Feng, Yanjun Li, Jingyu Zhao, Li Yu, Ziyi Song (Nanjing University of Aeronautics and Astronautics, China) | Estimation of ejection speed of parachute shelter based on flow analysis |
11:35-11:50 | Oral | 058 | Yanjun Li, Zhen Liu, Ziyue Chen, Wei Wang (Nanjing University of Aeronautics and Astronautics, China) | Effect of the confined flow on concurrent-flow flame spread in microgravity |
11:50-12:05 | Oral | 050 | Yuxiang Fang, Xiande Fang , Xinyi Wang , Yeqi Qin (Nanjing University of Aeronautics and Astronautics, China) | Modelling of heat transfer coefficient of single-phase flow in spiral tubes |
12:05-13:30 Lunch | ||||
Afternoon, Chair: Prof. Bin Chen, Xi’an Jiaotong University, China | ||||
Time | Activity | PaperNo. | Presenter | Title |
13:30-14:00 | Keynote speech | K02 | Lixing Zhou (Tsinghua University, China) | Progress in validation of the SOM turbulent combustion model by DNS |
14:00-14:15 | Oral | 065 | Fan Wang, Minqi Zhang, Yudong Wang, Yuxuan Han, Jie Jin (Beihang University, China) | Kerosene duel swirl two phase combustion simulation with algebraic second order moment model |
14:15-14:30 | Oral | 059 | Ziqi Fu, Juli Fan (Nanjing University of Aeronautics and Astronautics, China) | Numerical study on drying process of lyoprotectant solutions |
14:30-14:45 | Oral | 007 | Hengyuan Wang, Fucheng Chang, Jiacheng Lou, Yeming Liu, Zongrui Feng , Huixiong Li (Xi’an Jiaotong University, China) | Numerical simulation of heat transfer deterioration and heat transfer non-uniform characteristics in helically-coiled tube with large heat flux |
14:45-15:00 | Oral | 039 | Yuanliang Xie, Yi Zhou (Nanjing University of Science and Technology, China) | Relevance of the viscous superlayer to pure shear layers in a temporally evolving turbulent planar jet |
15:00-15:15 | Oral | 052 | Pucheng Yang, Xiande Fang, Qiumin Dai (Nanjing University of Aeronautics and Astronautics, China) | Modelling of drag coefficient of stratospheric airship |
15:15-15:30 | Oral | 043 | Xiaoyang Li, Xiaohui Lin,Changyue Xu , Zhuopei Li (Nanjing University of Aeronautics and Astronautics, China) | Numerical simulation of transient heat load of a sightseeing airship cockpit |
15:30-15:45 | Break | |||
Chair: Prof. Fang Wang, Beihang University, China | ||||
15:45-16:00 | Oral | 002 | Shuqin Xue, Jing Yin, Shuyan Chen, Dongqing Zhu, Zhifu Zhou*, Bin Chen (Xi’an Jiaotong University, China) | Experimental and numerical investigation of the evaporation characteristics of ethanol droplet in complex conditions |
16:00-16:15 | Oral | 030 | Liuyun Lu, Huixiang Wu, Xiaohui Lin, Changyue Xu (Nanjing University of Aeronautics and Astronautics, China) | The flow patterns in the pipeline with twisting column |
16:15-16:30 | Oral | 055 | Yevhenii Shkvar , Jonas Kandume (Zhejiang Normal University, China) | Numerical and experimental study of the vortical flow generated by ellipsoidal dimples |
16:30-16:45 | Oral | 003 | Galina Ya. Dynnikova (Lomonosov Moscow State University, Russia) | High-Resolution meshless simulation of a viscous incompressible fluid flow around an elliptical cylinder |
16:45-17:00 | Oral | 014 | Musfequs Salehin, A. B. M. Toufique Hasan (BSMR Aviation and Aerospace University, Bangladesh) | Effects of airfoil thickness on aerothermal characteristics at supersonic transitional rarefied conditions |
17:00-17:15 | Oral | 017 | Alexandra Kolganova, Ilia Marchevsky, Evgeniya Ryatina (Bauman Moscow State Technical University, Russia) | Fast algorithm for a boundary integral equations solving in 2D flows simulation using vortex particle method |
17:15-17:30 | Oral | 009 | Zhiyuan Shan, Xiaoyuan Sun, Feng Deng (Nanjing University of Aeronautics and Astronautics, China) | Numerical study on the hydrodynamic characteristics of skipping stones |
17:30-17:45 | Oral | 063 | Nikolai Kornev, Sina Samarbakhsh , Pascal Anschau (University of Rostock, Germany) | Vortex structures of ships and their influence on vibration of energy saving ducts |
18:00-20:00 | Buffet |
Wednesday, Oct. 18, 2023 | ||||
Building A18-529, Nanjing University of Aeronautics and Astronautics | ||||
Morning, Chair: Prof. Alan Jeffrey Giacomin, Queen’s University, Canada | ||||
Time | Activity | Paper No. | Corresponding author | Title |
8:30-9:10 | Keynote speech | K03 | Nikolai Kornev (University of Rostock, Germany) | VπLES- hybrid vortex and grid based method for simulation of turbulent flows at high Re numbers |
9:15-9:30 | Oral | 038 | Yahao Song, Zhi Wei, Changyue Xu, Ke Liu (Nanjing University of Aeronautics and Astronautics, China) | Detached eddy simulation of transonic buffet on a supercritical airfoil |
9:30-9:45 | Oral | 040 | Nailiang Zhuang, Yangyang Li, Zhiling Guan, Hetong Li, Xiaobin Tang (Nanjing University of Aeronautics and Astronautics, China) | Numerical simulation of structural evolution of secondary flow in variable curvature spiral tube of steam generator |
9:50-10:05 | Oral | 066 | Ziyue Chen, Yanjun Li, Li Yu (Nanjing University of Aeronautics and Astronautics, China) | Optimization of parafoil design based on Isight |
10:05-10:20 | Oral | 020 | Fang Wang , Yudong Wang , Yunfan Wang, Min Zhang (Beihang University, China) | The two phase turbulence combustion simulation with IBM method and applications |
10:20-10:35 | Oral | 046 | Yiyang Guo, Xiande Fang, Xinyi Wang, Yeqi Qin (Nanjing University of Aeronautics and Astronautics, China) | Analysis of friction factor correlations of single-phase flow in spiral tubes |
10:35-10:50 | Break | |||
Chair: Prof. Changyue Xu, Nanjing University of Aeronautics and Astronautics, China | ||||
10:50-11:05 | Oral | 010 | Yanxin Yin, Ruiyang Lu, Jianxin Liu, Zhangfeng Huang (Tianjin University, China) | Real gas effects on receptivity to roughness in hypersonic swept blunt flat-plate boundary layers |
11:05-11:20 | Oral | 064 | Shuyue Liang, Weijian Chen, Hui Ma, Guangya Zhu, Yan Wu (Nanjing University of Aeronautics and Astronautics, China) | Numerical simulation of vortex oil filter performance improvement |
11:20-11:35 | Oral | 070 | Shengfang Lu, Weijian Chen, Dalin Zhang, Guangya Zhu, Penglei Zhang (Nanjing University of Aeronautics and Astronautics, China) | Research and optimization design of aircraft windshield hot gas rain removal system |
11:35-11:50 | Oral | 053 | Wenbo Yang, Xuehao Sang, Bin Chen (Xi’an Jiaotong University, China) | Numerical Simulation of R410A closed-loop spray cooling system |
11:50-12:05 | Oral | 049 | Chong Li, Xiande Fang (Nanjing University of Aeronautics and Astronautics, China) | Numerical analysis on flow pattern of flow boiling in a horizontal tube |
12:05-13:30 Lunch | ||||
Afternoon, Chair: Prof. Zhifu Zhou, Xi’an Jiaotong University, China | ||||
Time | Activity | Paper No. | Corresponding author | Title |
13:30-14:10 | Keynote speech | K04 | New Tze How (Nanyang Technological University, Singapore) | Recent insights on vortex rings colliding with solid boundaries and density interfaces |
14:10-14:25 | Oral | 031 | Yulia Izmailova, Ilia Marchevsky, Kseniia Sokol, Evgeniya Ryatina (Bauman Moscow State Technical University, Russia) | The VM2D source code for incompressible flow simulation around airfoils using vortex methods: innovations and approaches for FSI problems |
14:25-14:40 | Oral | 061 | Xiaohui Lin , Bin Wang, Zongru Li, Changyue Xu (Nanjing University of Aeronautics and Astronautics, China) | Numerical simulation of the compressible flow past a tabbed cylinder involving yawed angle |
14:45-15:00 | Oral | 062 | T. H. New , R. You and W. L. Chan (Nanyang Technological University, Singapore) | Standoff shock and shear layer vortex behaviour in supersonic impinging jets |
15:00-15:15 | Oral | 011 | Gen Li, Yiling Zhao, Xiande Fang, Yuetian Liu (Chongqing Jiaotong University, China) | Study on flow boiling heat transfer characteristics of Al2O3-water nanofluids under low-hypergravity conditions |
15:15-15:30 | Oral | 032 | Daren Zheng, Pei Li, Jianhong Sun, Changyue Xu, Zhi Sun (Nanjing University of Aeronautics and Astronautics, China) | Thermal performance enhancement of a rectangular channel with dual grooves |
15:30-15:45 | Break | |||
Chair: Prof. Ilia Marchevsky, Bauman Moscow State Technical University, Russia | ||||
15:45-16:00 | Oral | 037 | Gennadii Voropaiev, Yevhenii Shkvar, Yaroslav Zagumennyi (Institute of Hydromechanics of NASU, Ukraine) | Vortex formation by oscillating bodies |
16:00-16:15 | Oral | 026 | Taketo Haruki, Shogo Ando , Tetsuya Yagi , Hiroshi Amano , Yasumasa Iwatani , Kotaro Takamure , Tomomi Uchiyama (Nagoya University, Japan) | Particle collection characteristics of a prismatic two-stage electrostatic precipitator |
16:15-16:30 | Oral | 036 | Daisuke Kobayashi , Kotaro Takamure, Tomomi Uchiyama (Nagoya University, Japan) | Visualized measurement of the wake of a sphere having a uniaxial through-hole |
16:30-16:45 | Oral | 028 | D. Kotsopoulos, K. Kalogiannis, A. Romeos, A. Giannadakis, K. Perrakis, Th. Panidis and B. Chen (University of Patras, Greece) | Refrigerant spray cooling of electronics |
16:45-17:00 | Oral | 018 | Masato Ogawa and Kota Fukuda (Tokai University, Japan) | Fundamental study on physics informed neural network model based on vortex methods |
17:00-17:15 | Oral | 035 | Xiande Fang, Zhiqiang He, Xinyi Wang, Yeqi Qin (Nanjing University of Aeronautics and Astronautics, China) | New correlation of heat transfer coefficient for saturated flow boiling in helically coiled tubes |
17:15-17:30 | Oral | 068 | Chenlu Wang, Jianhong Su, Hao Liu, Changyue Xu, Daren Zheng (Nanjing University of Aeronautics and Astronautics, China) | Aerodynamic design of airfoil in ground effect based on the conditional generative adversarial network |
17:30-17:45 | Oral | 034 | Yaroslav Demchenko, Vasily Vedeneev, Alexander Zubkov (Lomonosov Moscow State University, Russia) | Torsional aero-elastic oscillations of an elastically mounted circular cylinder |
18:00-20:00 | Banquet |
Thursday, Oct. 19, 2023 | ||||
Building A18-529, Nanjing University of Aeronautics and Astronautics | ||||
Morning, Chair: Prof. Daniel New Tze, Nanyang Technological University, Singapore | ||||
Time | Activity | Paper No. | Corresponding author | Title |
8:30-9:10 | Keynote speech | K05 | Chaoqun Liu ( University of Texas at Arlington, USA) | Liutex – unique and rigorous definition and identification of vortex |
9:15-9:30 | Oral | 033 | Ilia Marchevsky, Georgy Shcheglov, Sergey Dergachev (Bauman Moscow State Technical University, Russia) | Three-dimensional meshless vortex loops method for hydrodynamic loads estimation acting on structures in the flow |
9:30-9:45 | Oral | 001 | Zhanru Zhou, Shenghong Huang , Huanchun Huang , Menglai Jiang , Zhiwei Pan (University of Science and Technology of China, China) | A new correlation of the subcooled flow boiling heat transfer coefficient for hybrid micro/nanostructured surfaces with high heat flux incidence |
9:50-10:05 | Oral | 015 | Yingjie Zhao, Guozeng Feng, Rui Hong, Yueteng Guo, Xiaoyong Zuo (Jiangsu University of Science and Technology, China) | Energy saving design of heating coil and heating system for liquid cargo cabin of 15000DWT asphalt carrier |
10:05-10:20 | Oral | 027 | Lincheng Xu, Gang Wang (Northwestern Polytechnical University, China) | Performance analysis of anguilliform swimming fish with different body sections in large eddy simulations using adaptive Cartesian grids |
10:20-10:35 | Oral | 048 | Ranran Huang, Jie Wu (Huazhong University of Science and Technology, China) | Measurement of the hypersonic turbulent boundary layer using two-point focused laser differential interferometry |
10:35-10:50 | Break | |||
Chair: Prof. Xiaowei Zhu, Beihang University, China | ||||
10:50-11:05 | Oral | 016 | Chunqi Wang, Kotaro Takamure, Tomomi Uchiyama (Nagoya University, Japan) | Experimental Investigation on Jet Issuing from Two-Dimensional Nozzle Equipped with Cut Airfoil of NACA0036 |
11:05-11:20 | Oral | 060 | Yalei Bai, Min Zheng (Nanjing University of Aeronautics and Astronautics, China) | Numerical study of a flapping foil in energy harvesting with suction and blower based control |
11:20-11:35 | Oral | 012 | Pengcheng He | The new combined waves theory |
11:35-11:50 | Oral | 024 | Yi Liu, Xiangwei Lin, Dongqing Zhu, Zhifu Zhou, Bin Chen (Xi’an Jiaotong University, China) | Numerical study on heat transfer of refrigerant R410A flash spray cooling |
11:50-12:05 | Oral | 045 | Yanting Zhang, Nailiang Zhuang (Nanjing University of Aeronautics and Astronautics, China) | Two-phase flow pattern transition in variable curvature helical coil |
12:05-13:30 Lunch | ||||
Afternoon, Chair: Prof. Yanjun Li, Nanjing University of Aeronautics and Astronautics, China | ||||
Time | Activity | Paper No. | Corresponding author | Title |
13:30-13:45 | Oral | 067 | Zihao Zhang, Weijian Chen, Qinyang Li, Zhiyuan Qu, Dalin Zhang (Nanjing University of Aeronautics and Astronautics, China) | Engine lip jet impingement and film heating coupled anti-icing structure |
13:45-14:00 | Oral | 005 | Guohua Li *, Jie Gong, Ran Liu, Zijuan Wang (Beijing Institute of Spacecraft Environment Engineering, China) | Numerical calculation of gas-liquid two-phase flow in Tesla valve |
14:00-14:15 | Oral | 023 | Bin Wang, Yahao Song, Xiaohui Lin, Changyue Xu (Nanjing University of Aeronautics and Astronautics, China) | Numerical investigation on transonic buffet of supercritical airfoils |
14:15-14:30 | Oral | 004 | Yisheng Gao, Jiajun Long, Fei Qin (Nanjing University of Aeronautics and Astronautics, China) | Implementation of liutex-based direct integrated field inversion and machine learning framework in PHengLEI solver |
14:30-14:45 | Oral | 057 | Helei Zhang, Xiande Fang, Yuying Zhang, Huixiang Wu (Nanjing University of Aeronautics and Astronautics, China) | Investigation on gas-liquid-solid three-phase flow characteristics in aircraft wastewater pipes |
14:45-15:00 | Oral | 022 | Yukun Yong, Jianhong Sun, Yang Tao, Hao Liu, Changyue Xu, Daren Zheng (Nanjing University of Aeronautics and Astronautics, China) | Numerical investigation of active control of airfoil involving ground effect |
15:00-15:15 | Oral | 029 | Xiaoyingjie Huang, Jiabao Chen,Yan Wang (Nanjing University of Aeronautics and Astronautics, China) | Rotated lattice boltzmann flux solver based on adaptive mesh refinement for numerical simulation of compressible flows with complex shock structures |
15:15-15:30 | Oral | 019 | Yuanyuan Wang, Chengyue Wang (Nanjing University of Science and Technology, China) | Investigation on the vortex dynamics of jellyfish-like flapping wings based on particle image velocimetry |
15:30-15:45 | Oral | 013 | Quanzheng Li, Xiaoyu Sun, Gang Wang (Northwestern Polytechnical University, China) | Detached-eddy simulation of the vortex system on the high-lift common research model |
18:00-20:00 | Buffet |
- Tips:
Keynote speeches are limited to 40 minutes which includes:
35 minutes for the report if 40 minutes, and 25 minutes for the report if 30 minutes
5 minutes for questions/discussions
Presentations are limited to 15 minutes which includes:
12 minutes for the report
3 minutes for questions/discussions
Keynote
1. Recent Advances in Polymer Viscoelasticity from General Rigid Bead-Rod Theory
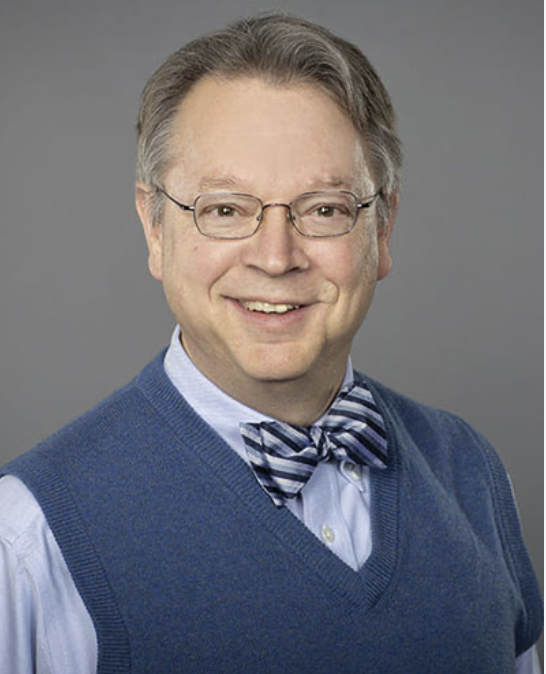
Editor-in-Chief, Physics of Fluids (IF 4.980)
Corporate Secretary, AIP Publishing
NSERC Tier 1 Canada Research Chair in Physics of Fluids
Professor of Chemical Engineering
Professor of Mechanical and Materials Engineering
Professor of Physics, Engineering Physics and Astronomy
Queen’s University, Canada
Title: Recent Advances in Polymer Viscoelasticity from General Rigid Bead-Rod Theory
Abstract: One good way to explain the elasticity of a polymeric liquid, is to just consider the orientation distribution of the macromolecules. When exploring how macromolecular architecture affects the elasticity of a polymeric liquid, we find general rigid bead-rod theory to be both versatile and accurate. This theory sculpts macromolecules using beads and rods. Whereas beads represent points of Stokes flow resistances, the rods represent rigid separations. In this way, how the shape of the macromolecule affects its rheological behavior in suspension is determined. Our work shows the recent advances in polymer viscoelasticity using general rigid bead-rod theory, including advances applied on the coronavirus. The coronavirus is always idealized as a spherical capsid with radially protruding spikes. However, histologically, in the tissues of infected patients, capsids in cross section are elliptical, and only sometimes spherical. This capsid ellipticity implies that coronaviruses are oblate or prolate or both. We call this diversity of shapes, pleomorphism. Recently, the rotational diffusivity of the spherical coronavirus in suspension was calculated, from first principles, using general rigid bead-rod theory. We did so by beading the spherical capsid, and then also by replacing each of its bulbous spikes with a single bead. In this paper, we use energy minimization for the spreading of the spikes, charged identically, over the oblate or prolate capsids. We use general rigid bead-rod theory to explore the role of such coronavirus cross-sectional ellipticity on its rotational diffusivity, the transport property around which its cell attachment revolves. We learn that coronavirus ellipticity drastically decreases its rotational diffusivity, be it oblate or prolate.
Short Vita: Dr. Alan Jeffrey Giacomin is a retired Professor of Chemical Engineering, Mechanical and Materials Engineering and Physics, Engineering Physics and Astronomy at Queen’s University at Kingston in Canada where he holds the titles Tier 1 Canada Research Chair in Physics of Fluids. Prof. Giacomin has been serving as sole Editor-in-Chief of Physics of Fluids since 2016. He is an adjunct professor at University of Nevada, Reno in USA and an adjunct professor of Peking University, Beijing in China. Prof. Giacomin earned his bachelor’s and master’s degrees in Chemical Engineering from Queen’s University in Kingston, before joining the Research Division at DuPont Canada. He then earned a PhD in Chemical Engineering from McGill. He joined the Mechanical Engineering faculty at Texas A&M University. He has been Professor of Mechanical Engineering at the University of Wisconsin, where for twenty years he chaired its Rheology Research Center. He has held visiting professorships at McGill University, the University of Sherbrooke, the Swiss Federal Institute of Technology, the Paris School of Mines, the National University of Singapore, Chung Yuan University near Taipei, Yunlin University, in southern Taiwan, and Shandong University in mainland China. He is Past-President of the Canadian Society of Rheology, and a former President of The Society of Rheology. His research interests are dynamics of polymeric liquids, plastics and polymer processing, non-Newtonian fluid mechanics; rheometry, nonlinear viscoelasticity, large-amplitude oscillatory shear flow, thermoforming; pipe extrusion, die drool, injection molding, melt index, and durometry. Prof. Giacomin has received many awards and honors, and he has published more than 370 academic papers, including 180 refereed journal papers.
2. Liutex and Third Generation of Vortex Definition and Identification
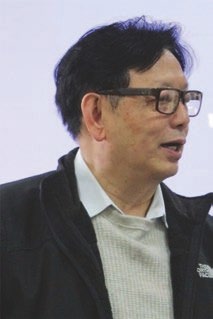
Title: Liutex and Third Generation of Vortex Definition and Identification
Abstract: Vortex is intuitively recognized as the rotational/swirling motion of fluids. However, a rigorous mathematical definition for vortex was absent for centuries. Liutex is a new physical quantity to represent fluid rotation or vortex, proposed by Liu et al. at University of Texas at Arlington (UTA) in 2018. Since then, they have published over 40 SCI journal papers including 13 PoF papers, many of them are top cited papers. Three professional books on Liutex have been published by Bentham in 2020, Elsevier in 2020 and Springer in 2021. There are three generations of vortex identification methods in history. In 1858, Helmholtz first defined vortex as vortex tube composed by the so-called vortex filaments. It is classified as the first generation of vortex identification that vortex is defined as vorticity tubes. Science and engineering applications have shown that the correlation between vortex and vorticity is very weak, especially in the near-wall region. During the past four decades, many vortex identification criteria including Q-, Lambda_2, Lambda_Ci methods have been developed, which are classified as the second generation of vortex identification. They are all based on the eigenvalues of the velocity gradient tensor. However, they are all scalars and thus strongly threshold-dependent. In addition, they are all obviously contaminated by stretching and shearing. Liutex, as the third generation of vortex definition and identification, is defined as a vector which uses the real eigenvector of velocity gradient tensor as its direction and twice the local angular velocity of the rigid rotation as its magnitude. The major idea of Liutex is to extract the rigid rotation part from fluid motion to represent vortex. After that, a number of new vortex identification methods have been developed by Liu and the UTA Team including Liutex vector, Liutex vector lines, Liutex tubes, Liutex iso-surface, Liutex-Omega methods, Objective Liutex and, more recently, Liutex Core Line methods. Liutex-Omega method is very popular and Liutex-Core-Line method is unique and threshold-free. The Liutex definition, Principal Coordinate, Principal Decomposition in Principal Coordinate, vorticity RS decomposition, velocity gradient tensor UTA R-NR decomposition, and Principal Decomposition of velocity gradient tensor in Cartesian coordinates, proposed by Liu et al., integrate the Liutex theoretic system and pave the foundation for new fluid kinematics, new vortex science and new turbulence research. A new fluid kinematics has been derived in details and a new fluid dynamics is ongoing.
Short Vita: Dr. Chaoqun Liu received both BS (1968) and MS (1981) from Tsinghua University, Beijing, China and Ph. D. (1989) from University of Colorado at Denver, USA. He is currently the Tenured and Distinguished Professor and the Director of Center for Numerical Simulation and Modeling at University of Texas at Arlington, Arlington, Texas, USA. He has worked on high order direct numerical simulation (DNS) and large eddy simulation (LES) for flow transition and turbulence for over 30 years since 1990. As PI, he has been awarded by NASA, US Air Force and US Navy with 50 federal research grants of over 5.7 million US dollars in the United States. He was Chairman of the First and Third AFOSR International Conference on DNS/LES in 1997 and 2001. He has published 14 professional books, 129 journal papers and 152 conference papers. He is the founder and major contributor of Liutex and the third generation of vortex definition and identification methods including the Omega, Liutex/Rortex, Modified Liutex-Omega, Liutex-Core-Line methods, RS vorticity decomposition and UTA R-NR velocity gradient tensor decomposition. He is also the founder of the new fluid kinematics which is the foundation for new fluid dynamics.
3. VπLES- hybrid vortex and grid based method for simulation of turbulent flows at high Re numbers
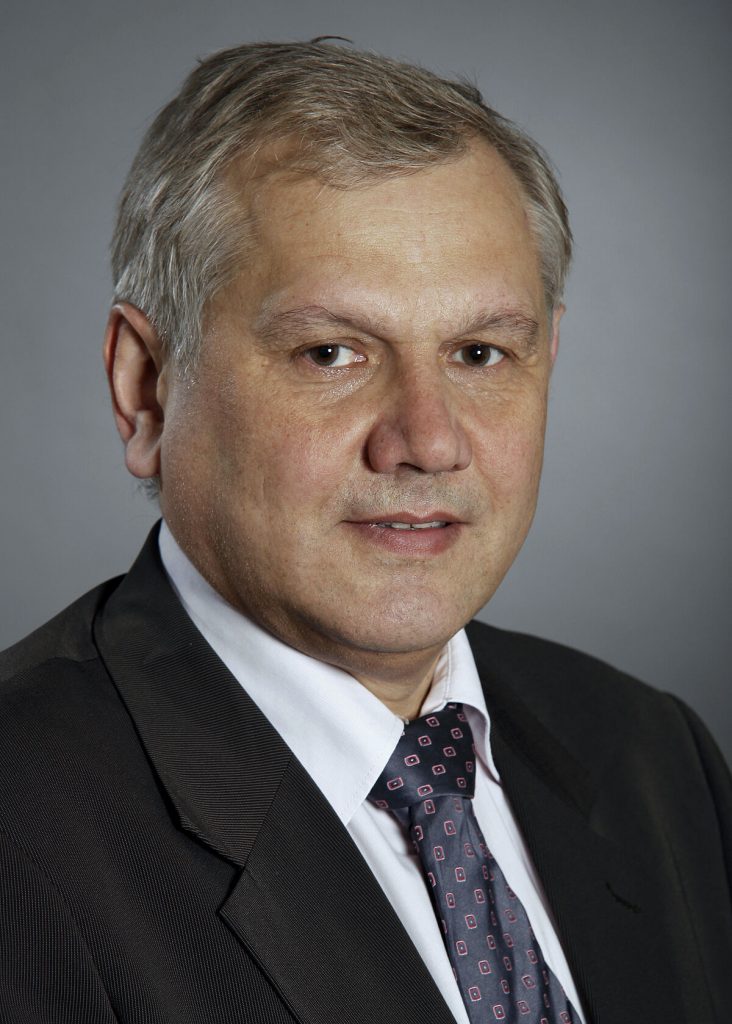
Title: VπLES- hybrid vortex and grid based method for simulation of turbulent flows at high Re numbers
Abstract: The lecture presents development and validation of a new computational fluid dynamics (CFD) method using a combination of grid-free (Vortex Particle Method, VPM) and grid-based (Finite Volume Method) techniques. The Lagrangian particle method VPM is suitable for modeling of fine and fast flow structures whereas the grid-based techniques like FVM have strong advantages in the modeling of large-scale motions. A fundamental assumption of this novel approach is the decomposition of any physical quantity into the grid based (large scale) and the fine scale parts, whereas large scales are resolved on the grid and fine scales are represented by particles. Dynamics of large and fine scales is calculated from two coupled transport equations one of which is solved on the grid whereas the second one utilizes the Lagrangian grid free VPM. The method is implemented into OpenFOAM in a parallel mode and can be used for computations of complex flows on arbitrary unstructured grids. The performance of the method is illustrated for the isotropic homogeneous turbulence, free jets, wall bounded flows and mixing.
Short Vita: Prof. Kornev is the head of the Chair of Modelling and Simulation at the Rostock University since 2010. He studied Fluid Mechanics at the Marine Technical University in St. Petersburg, Russia and received his PhD (Candidate of Science) and habilitation (Doctor of Science) degrees from the same university in, respectively, 1988 and 1998. Since 2001 he is working at the Rostock University dealing with fluid dynamics, turbulence research, Computational Fluid Dynamics, mixing, ship hydromechanics and heat transfer. One of his research topics is the development and application of the computational vortex methods.
4. Recent insights on vortex rings colliding with solid boundaries and density interfaces
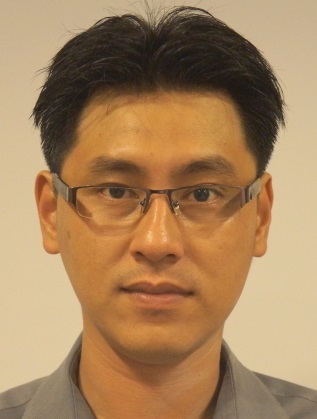
Title: Recent insights on vortex rings colliding with solid boundaries and density interfaces
Abstract: As highly fundamental flow entities, vortex rings have always intrigued researchers with their simplicity and elegance, not to mention how they shed light upon more complex flow phenomena. In particular, interactions between vortex rings and solid boundaries, as well as non-solid density interfaces, have roused much interest among researchers in recent years. This is in no small part due to how such flow scenarios reveal much about vortex-boundary layer and vortex-vortex interactions, which could in turn lead to formulations of novel concepts that could prove to be useful for mitigating flow control issues. In this presentation, several vortex ring collision, and hence interaction, scenarios studied recently through experimental and numerical means will be elaborated in terms of their key flow and vortex dynamics. These scenarios will include those associated with various solid geometries and density interfaces, whereby the surprisingly wide range of interaction outcomes demonstrate just how versatile vortex rings are when it comes down to understanding flow mechanisms that bridge real-world applications.
Short Vita: Dr. Daniel T. H. New graduated from the National University of Singapore (NUS) with a PhD in Mechanical Engineering in 2004. He worked in Temasek Laboratories, NUS as an Associate Scientist and later as a Research Scientist, before he ventured into combustion research at the University of Texas, Arlington as a post-doctoral researcher. He joined the University of Liverpool, UK as a Lecturer in 2005 and worked on jet flow control strategies with support from EPSRC UK and The Royal Society. He joined Nanyang Technological University, Singapore in 2010 where he is currently an Associate Professor and was the Associate Chair (Students) of the School of Mechanical and Aerospace Engineering from 2016 to 2018. He was also a Visiting Professor at ONERA and Monash University in 2018 and 2019 respectively. His current research interests include vortex-ring and jet vortex dynamics, Light-field camera-based PIV technique, bluff body flows and supersonic flows, among others. Together with his collaborators, he has edited three books, published nine book chapters, about a hundred journal papers and more than eighty conference articles.